Induced Resistance for Plant Health
Meirav Leibman-Markus, Rupali Gupta, and Maya Bar
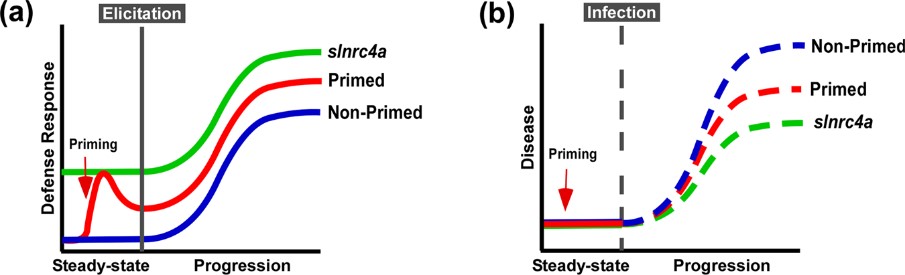
*Department of Plant Pathology and Weed Research, ARO, Volcani Institute, Rishon Leziyon, Israel.
mayabar@volcani.agri.gov.il
https://mayapiff.wixsite.com/barlab
Plants are sessile and therefore cannot escape pathogens, arthropods or abiotic stress. They defend themselves through physical and chemical barriers, e.g., leaf wax, cell wall, and inhibitory compounds such as antibiotic, that contribute to their survival 1. In order to cope with environmental stress conditions (both biotic and abiotic), plants developed sophisticated mechanisms for sensing different stresses and adapting to them by rapid, dynamic and complex physiological changes. Ideally, these changes will lead to increased resistance to existing stress, without compromising plant fitness. A comprehensive understanding of the molecular and physiological mechanisms underlying induced disease-resistance will lead to a deeper understanding of stimulus sensing and downstream immune-signaling. This will hopefully enable improved and sustainable management of plant diseases in agriculture ecosystems or environments.
Figure 1:CK reduces disease symptoms of necrotrophic and biotrophic fungi in tomato.M82 tomato plants were treated with indicated concentrations of CK (6-BAP). Twenty four hours later, plants were inoculated with 10 µl of B. cinerea spore solution (106 spores/mL, a-f) or O. neolycopersici (104spores/mL, g). a-e. Representative pictures of B. cinerea infection in leaves treated with different CK concentration, as indicated. Bar: 1 cm. f.Lesion area was measured on whole plants 5 days after B. cinerea inoculation. g. Leaf disease coverage was calculated 10 days after O.neolycopersici inoculation.
Adapted from Gupta et al., 20201.
Induced resistance (IR) is increased sensitivity and responsiveness to stress, preconditioned by prior infection or exposure to stimuli, which primes enhanced resistance. It is an integral part of the induced immunity. The plant takes protective measures against potential attackers, alerting its defense system for a faster and / or stronger response in the future. IR is effective against subsequent challenge by various pathogens and pests as well as abiotic stress. There are two forms of IR, systemic acquired resistance (SAR) and induced systemic resistance (ISR)2.
Systemic acquired resistance (SAR) is a form of IR that is activated throughout the plant after exposure to elicitors from virulent, avirulent, or non-pathogenic microbes, or artificial chemical stimuli such as chitosan or salicylic acid (SA). The resistance commences in the vicinity of the initial infection site (localized acquired resistance (LAR)), and spreads to distant tissues throughout the plant, hence, systemic acquired resistance (SAR). Both localized and systemic- acquired resistance are characterized by an increase in pathogen-related (PR) proteins that may also have antimicrobial properties and contribute to resistance.The signaling mechanism enabling distal SAR has not yet been fully elucidated, but the main players are believed to be salicylic acid (SA), and derivatives of SA such as methyl salicylate (MeSA).
Induced systemic resistance (ISR) is a resistance mechanism in plants that is activated by beneficial microorganisms such as rhizosphere bacteria (rhizobacteria) and fungi. Plant growth promoting rhizobacteria and fungi (PGPR and PGPF, respectively) can create a chemical based symbiosis with plants that includes a stimulus for the priming of ISR. The signaling pathway of ISR is jasmonic acid (JA) and ethylene (ET) dependent. ISR does not necessarily lead to the eradication or inhibition of the invading pathogen, but rather leads to increased physical or chemical barriers in the host plant.
In our lab we study various stimuli that prime IR, including, through an application of chemicals or biologicals, alteration of physical conditions, and genetic manipulation.
Plant hormones play a pivotal role in plant immunity in general and in IR in particular. The “classical” immune-hormones: SA, JA and ET, are known for differentially regulating immunity. SA is considered to mediate defense against biotrophs and induce SAR, while JA mediates the signaling pathway of necrotrophs and the induction of ISR, yet emerging data point to a crosstalk and merging points between two pathways. In recent years, the involvement of other hormones, such as auxin, gibberellic acid (GA), abscisic acid (ABA), and cytokinins (CKs), in biotic stresses has been recognized. In a recent work, we demonstrated that exogenous CK can act as a chemical stimuli for IR, ameliorating disease outcomes of the tomato necrotrophic fungus Botrytis cinerea (Bc) and biotrophic fungus Oidium neolycopersici in a dose dependent manner (Figure 1) and enhancing immune responses3. Distinctions between SAR and ISR are not always clear-cut and overlaps and/or co-activation between these pathways have been reported. Our work suggests that in tomato, CK activates systemic resistance that requires SA signaling. However, evidence of overlap between SAR and ISR also exists in the context of CK, as we found that CK activates classical ISR genes, and requires ET, though not JA, to induce pathogen resistance. This is perhaps not surprising, given that CK signaling was reported to be upstream of ET.
Figure 2. Gram-positive bacilli isolated promoted disease resistance.M82 tomato plants were pre-treated with indicated bacteria, and subject to further experiments 3 days later. a. Plants were inoculation with B. cinerea (106spores/mL) and lesion area was measured 3 days after inoculation. b. Plants were challenged with the elicitor,flg-22 (flagellin peptide 22). ROS production was measured using the HRP luminol method and summarized to total RLU (Relative Luminescence Units).
Adapted from Gupta et al., 2022 2.
The emerging role of CK as an immune-hormone, and its ability to act as a chemical stimuli for the induction of IR, led us to investigate its effect on the plant microbiome. We were able to show that pre-treatment with certain gram-positive bacilli isolates, isolated from the phyllosphere of high CK genotypes, led to an immune-induction and increased resistance. Plants pretreated with the bacillus isolates displayed increased pathogen resistance to several pathogens, e.g., Bc (Figure 2a) and increased immune responses, e.g, flg-22 mediated immunity (Figure 2b). Overall, our results indicate that one mode of action of the phyllosphere bacterial isolates in disease prevention is via host immunity activation of IR4.
Figure 3. Root zone warming reduces gray mold disease. M82 tomato plants were subjected to RZW to 28ºC for 7 days and then inoculated with B. cinerea mycelia. Representative photos of plants that did not receive the RZW treatment (Mock, a) and plants that received the RZW treatment (b), 3 days post inoculation. c. Disease progression was monitored for 6 days by measuring lesion area.
Adapted from Gupta et al., 2021 3.
Apart from chemical and biological stimuli, alteration of physical conditions can act as a trigger for the induction of immunity as well. Given the possible implications for combinatorial stress resistance and pathogen control under temperature stress, we examined root zone warming (RZW) as a physical inducer of systemic disease resistance in tomato. We were able to decipher components of the molecular mechanisms underlying RZW-induced resistance. We have shown that RZW activates immunity in tomato, increasing the expression of defense genes and inducing defense responses. This activated immunity results in systemic disease resistance to plant pathogens of different lifestyles (biotrophic and necrotrophic), including botrytis (Figure 3), indicating that it is a mechanism common to several biotic signaling pathways. Interestingly, using different hormone mutants, we found that RZW-induced immunity relies on the SA and ET signaling pathways5.
Figure 4. slnrc4a gain of function mutant presents enhanced resistance to diverse plant pathogens and pests. WT (cv M82) and slnrc4a plants were challenged with several pathogens and pests. Bacterial growth (CFU) was measured 3 days after inoculation with (a) X. campestris (105 CFU/mL) and (b) P. syringae (105 CFU/mL). c Representative images of P. syringae symptomatic leaves. Lesion area was measured 3 days after inoculation with (d) B. cinerea (106 spores/mL) (e) S. sclerotium (106 spores/mL). f Representative images of S. sclerotium symptomatic leaves. Infestation was determined by counting number of insects per leaf and measuring % of infected leaf area two-weeks after (g) B. tabaci and (h) T. absoluta exposure, respectively. i Representative images of T. absoluta symptomatic leaves. j O. neolycopersici infection was measured as percentage of infected leaf out of total leaf area. k Representative images of O. neolycopersici symptomatic leaves. Adapted from Pizarro et al., 2020 4.
Figure 5. Suggested model of immuno-elicitation and disease response. a. Plants deploy defense responses after immuno-elicitation (blue), enhanced by priming, triggering a stronger and/or faster response (red). According to our results, slnrc4, plant defense responses are pre-activated, leading to stronger responses to immuno-elicitation (green). b. Plant disease progression in natural conditions (blue), retarded when plants are pre-treated with priming agents (red). Disease progression is lower in slnrc4 due to its pre-activated basal condition, in a manner which may be superior to primed plants (green).
Adapted from Pizarro et al., 2020 4.
A more “basic” way to induce resistance, is by genetically modifying plant immunity. Using CRISPR-Cas9 technology, we created a targeted gain of function mutation in the immune receptor NRC4a. This genetic manipulation resulted in a stronger immune system output and increased disease resistance against a wide spectrum of pathogens and pests (Figure 4). The gain of function mutation lines exhibited higher elicitor-mediated defense responses and enhanced biotic resistance, suggesting that slnrc4a mutated lines are genetically immuno-activated6.
IR can be sensitized by priming, potentiating defensive capacity after sensing diverse stimuli. Our work suggests that genetically enhancing innate immunity may be agriculturally superior to priming strategies, with an upregulation in defense responses free of the requirement for priming agents, leading to better reproducibility. The geneticaly manipulated lines display higher levels of a variety of genetic and physiological defense parameters prior to infection or exposure to stimuli6. Interestingly, in slnrc4a lines, the upregulation of basal immunity parameters is constitutive, and they do not return to basal levels in the absence of pathogen attack, as occurs in classical priming in wild type (WT) plants (Figure 5).
1. Agrios, G. N. How plants defend themselves against pathogens. In Plant Pathology 207–248 (Elsevier, 2005). doi:10.1016/B978-0-08-047378-9.50012-9.
2. Kamle, M. et al. Systemic Acquired Resistance (SAR) and Induced Systemic Resistance (ISR): Role and Mechanism of Action Against Phytopathogens. 457–470 (2020) doi:10.1007/978-3-030-41870-0_20.
3. Gupta, R., Pizarro, L., Leibman-Markus, M., Marash, I. & Bar, M. Cytokinin response induces immunity and fungal pathogen resistance, and modulates trafficking of the PRR LeEIX2 in tomato. Mol. Plant Pathol.21, 1287–1306 (2020).
4. Gupta, R. et al. Cytokinin drives assembly of the phyllosphere microbiome and promotes disease resistance through structural and chemical cues. ISME J.16, 122–137 (2022).
5. Gupta, R. et al. Root zone warming represses foliar diseases in tomato by inducing systemic immunity. Plant. Cell Environ.44, 2277–2289 (2021).
6. Pizarro, L. et al. A gain of function mutation in SlNRC4a enhances basal immunity resulting in broad-spectrum disease resistance. Commun. Biol.3, (2020).